For Carbon Removal, Scaling is More Critical than Permanence
Why an understanding of carbon flux is essential to a viable carbon market
The first trees nearly froze the earth. They towered above the understory thanks to their evolutionary ability to produce lignin, which gives trees their compressive strength. When these first great trees fell, no organism had yet evolved that could digest lignin, as fungi and terrestrial herbivores do today. As a result, these trees lay buried deep underground until humans excavated them as the world’s first coal deposits. At Living Carbon, we aim to enable natural and scalable carbon removal on par with these ancient evolutionary events by enhancing photosynthesis and slowing the decay rate of plants. Three hundred million years from now, a similar story could be told about today; an era of carbon sequestration so massive the rocks will tell our story. How do we achieve this? Here’s a hint: every major ice age over geological history is associated with an evolution in photosynthesis, coupled with other contributing factors such as continental positions, ocean currents, and enhanced weathering. At Living Carbon, we believe that intentionally recruiting large-scale biospheric and geological processes to assist human effort holds the most potential to draw down carbon in the quantity and time frame we need, to preserve the world we know.
A concept often encountered in carbon removal is that of “permanence,” the idea that the quality of carbon removal is best measured by the duration of storage of removed carbon out of the atmosphere. We believe the carbon removal industry is increasingly becoming hyperfocused on permanence without adequately valuing scalability. Fluent in commodity markets, it is easier to grasp an idea of fixed exchange: one ton of carbon is bought, and one ton is sequestered forever. While durability is important, the permanent removal market will not grow to be large enough by 2050 to achieve gigaton-scale removals. Scalability is under-discussed in both carbon removal markets and public policy.
The miscorrelation of permanence with efficacy in carbon drawdown has serious implications, as noted in a recent United Nations publication on carbon removal:
“The permanence of being chemically fixed (e.g. in rocks or in geological storage) is physical permanence (or physical irreversibility) and has no economic value beyond the time horizon. If we were to value carbon storage independently of any time horizon of interest, 1 tCO2 removed and stored through carbon mineralization could be considered to have a value 100, 1000, or 10,000 times greater than the value of 1 tCO2 removed and stored for 100 years. This leads us to an absurd conclusion that we know is not true.” - United Nations Article 6.4 Supervisory Board; Removal Activities Under the Article 6.4 Mechanism, May 2023
Carbon removal is often thought of as the accumulation of a commodity in a storage vault, where the focus is primarily on the rate of return to the atmosphere as a determinant of quality. However, in our current situation, the rate of removal is far more important than the rate of return. The removal needs to happen within the next few decades to preserve our global biospheric systems. The production of carbon with thousand-year permanence is irrelevant if the scaling factor is a hundred times lower over a relevant time frame.
Using biotechnology, we are working with plants to increase the rate of photosynthesis, decrease the rate of decay, and clean soils made toxic by industrial activity. In addition to their improved rate of carbon uptake, our trees are more resilient to the negative effects of climate change; initial results show drought tolerance and resilience to increased temperatures, and they have the potential to grow on land where climate change is already degrading the ability of other trees to thrive.
Leveraging biological carbon drawdown enables us to avoid the high starting costs, and requirements for ongoing and intensive management seen in methods such as direct air capture. At Living Carbon, we focus on high-quality nature-based carbon projects by planting multiple species of trees, including our climate-smart seedlings, on land degraded by human activity. We are especially focused on abandoned minelands as sites of former fossil fuel extraction. The US alone has 133 million acres of reforestable land, capable of capturing 333 Mt of carbon annually. [Source: American Forests]
The Keeling Curve with Enhanced Biology
The Keeling Curve shows the ongoing change in the concentration of carbon dioxide in Earth’s atmosphere. It was named for the pioneering climatologist Charles David Keeling, who initiated the measurement program at the Mauna Loa Observatory in Hawaii. The Keeling Curve is beneficial for understanding climate change because it shows both the progressive increase in CO2 due to human emissions, and the annual seasonal fluctuation as biomass grows and decays throughout the year. There is variation due to changing rates of terrestrial photosynthesis over the year: the concentration of landmass in the Northern Hemisphere adds to the seasonal flux, with an annual drawdown during the Northern Spring and Summer and return due to decomposition in the Northern Winter.
Any carbon removal method will bend this curve downward in proportion to the removal rate, with the rate of return (re-release of CO2 into the atmosphere) subtracted. Enhanced biology offers significant near-term drawdown that can flatten the curve, expanding our window to reduce human emissions and develop complementary solutions.
Global Carbon Flux
Carbon flux is the continuous flow of carbon between Earth’s carbon pools, from the oceans to the atmosphere to geological formations and the bodies of living and dead organisms.

The Carbon Cycle: Carbon flows between each reservoir in an exchange called the carbon cycle, which has slow and fast components. Yellow numbers represent natural fluxes (in gigatons of carbon per year), red are human contributions in gigatons of carbon per year (or effects on natural fluxes from human contributions), and white numbers indicate carbon storage pools. [Source: NASA]
On average over the last decade, terrestrial plants absorb 123 gigatons (Gt) of carbon per year through photosynthesis, compared to human emissions of 9 Gt annually (NOAA). When we enhance the rate of photosynthesis, decrease the rate of decay, and regenerate degraded ecosystems, we are increasing the standing plant biomass pool (living plants) on Earth. Currently, the standing plant biomass pool is about 550 Gt, assuming a steady state, where photosynthetic drawdown is balanced by plant respiration and microbial decomposition of plant parts. In a given year, both these processes return approximately 60 Gt each of carbon to the atmosphere, with about 3 Gt added to the soil carbon pool (where 2,300 Gt currently reside).
In general, carbon flux between the atmosphere and the surface of the planet can be considered according to the "fast" (timescales of years to centuries) or "slow" (timescales of millennia to gigayears) carbon cycles. As expressed in the recent UN report, any carbon drawdown method must be considered relative to a relevant time horizon to be correctly evaluated for its efficacy.
“The value of removals, and indeed of emissions reductions or any climate action, is relative to our climate goals and our time horizon. If our goal was to tackle the next ice age, we might have set a time horizon of 25,000 years. But given the situation we are in, a time horizon of 100 years might be more appropriate. Of course, one could argue that it should be 200 or even 300 years.” - United Nations Article 6.4 Supervisory Board; Removal Activities Under the Article 6.4 Mechanism, May 2023
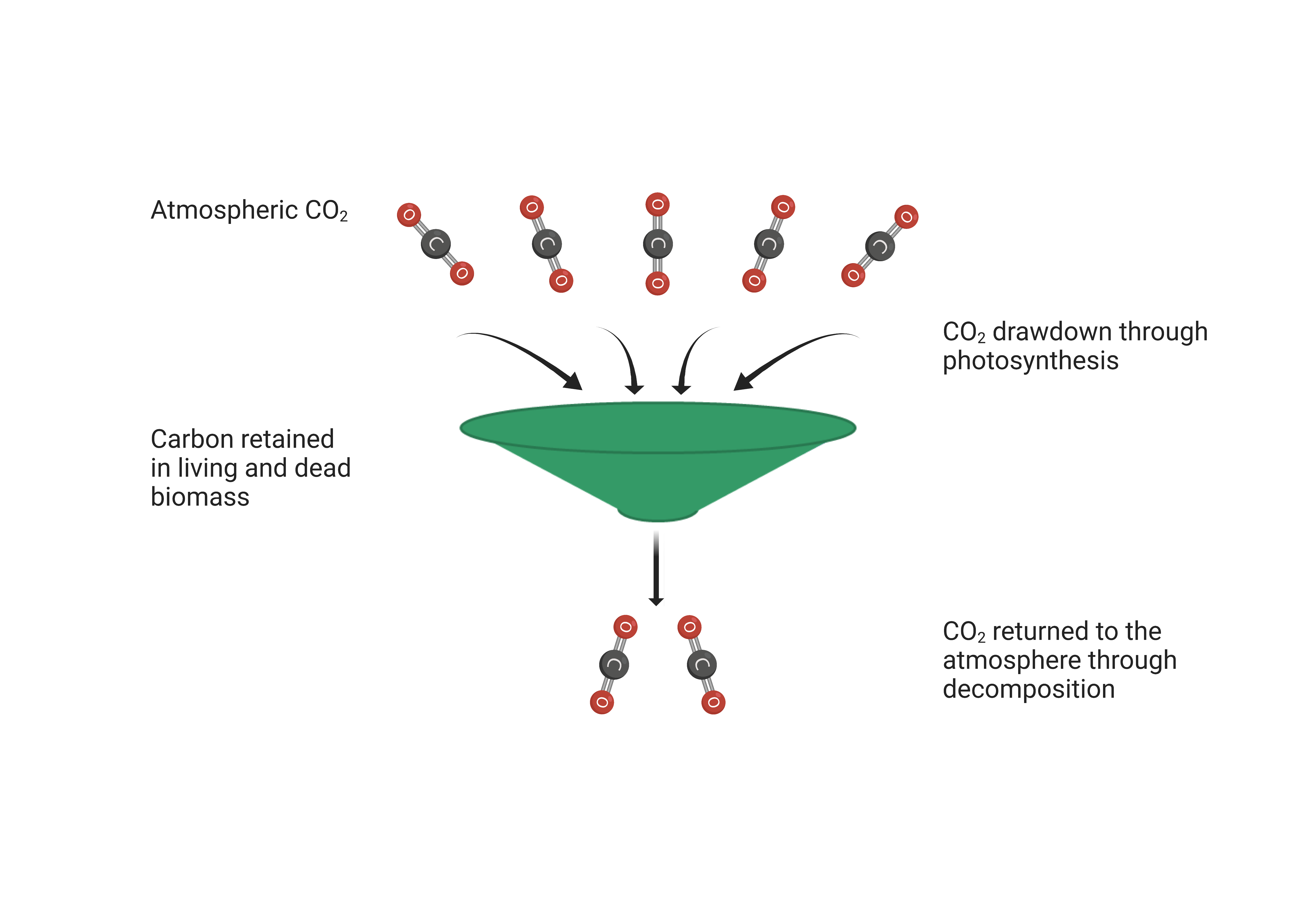
We can consider the biological carbon cycle as a funnel which carbon passes through. On one side, the intake of the funnel represents the fixation of atmospheric carbon dioxide. The body of the funnel represents the time carbon spends in living or dead biomass, and the output represents the return of carbon dioxide to the atmosphere through the respiration of decomposing organisms.
The rate of movement of carbon through the funnel is the carbon flux rate, which can be modeled by the differential in size between the input and output of the funnel. Analogizing carbon movement as a fluid flow in this way gives productive insights on how to best detain carbon as long as possible in the body of the funnel. Photosynthesis enhancement is analogous to increasing the width of the input, and decomposition resistance is analogous to constricting the output. Keeping this analogy in mind, let us look at how global carbon flux operates.
Human emissions result in an annual excess of about 4 Gt of carbon, averaged over the last decade and including the reduction in emissions in 2020 associated with the COVID-19 pandemic (source: NOAA), which accumulates in the atmosphere as roughly 14.68 Gt of CO2 per year. Atmospheric CO2 concentration is usually measured in parts per million (ppm), and 1 ppm represents an addition of 7.82 GT of CO2. This should therefore result in a year-on-year increase of about 1.88 ppm. The directly measured increase is more like 2.37 ppm/year, showing how our carbon cycle models still underestimate the degrading effects of human societies on the ability of the biosphere to retain fixed carbon. Future projections likely need to assume a baseline annual excess of just over 5 GT of carbon in the near-term. Efforts to absorb this excess and bend the curve of carbon accumulation in the atmosphere can take one of two general paths:
We have a few decades to rebalance the carbon cycle before global processes with immense energetic weight behind them (such as albedo changes, ocean current rerouting, and polar methane clathrate release) begin operating at scale and propel the planet into an ice-cap-free equilibrium, as they have many times before. Therefore, we need fast, scalable, and energetically favorable carbon solutions that increase biomass globally and enhance the ability of the biosphere to hold onto fixed carbon.
A permanent solution with high initial energy expenditure, such as direct air capture, offers the reassurance of a negligible rate of return. However, these solutions cannot take advantage of self-scaling processes in the manner of biological solutions, or of operation without continual energy input, as in enhanced weathering. We believe in all solutions on deck, including direct air capture, without focusing on permanence over scale as a measure of quality. DAC is especially efficient on emissions sources, where the high local concentration of carbon dioxide obviates the energetic inefficiency of removal from the general atmosphere. However, we would need 4 GT of carbon sequestered every year to reach net negative emissions with permanent sequestration alone. According to the International Energy Agency (IEA), “In the Net Zero Emissions by 2050 Scenario, direct air capture is scaled up to capture almost 60 MT CO2/year by 2030.” 60 MT of CO2 is equivalent to 16.4 MT of carbon, 0.0041% of what is needed. This is a drop in the bucket, so small that it makes no meaningful difference on the Keeling Curve.
We need significant near-term drawdown to flatten the curve and expand our window to both reduce human emissions and develop complementary solutions, including biomass injection, enhanced weathering, and direct air capture, to bring carbon flux as a whole into a state of net drawdown from the atmosphere to the land and oceans.
As an example of biological carbon flux in action, consider Pando, the ancient Aspen Grove, a tree species closely related to the poplars we are working with. Like many other aspen groves, Pando has persisted for millennia, but individual trees come and go over one or two centuries. Carbon is sequestered as new growth and incorporated into the root system as sugar is transported underground. While individual stems are temporary, the grove as a whole increases landscape-scale carbon storage over the longer term, as the persistent root system, where up to half the carbon is stored, continues to add biomass over millennia. Just as the grove can grow even while some trees die, so can we grow pools of carbon even if there is some release back to the atmosphere. We can grow these pools faster on less land with photosynthesis-enhanced and decomposition-resistant trees.
To increase the time that carbon remains out of the atmosphere, we can:
Achieving our climate goals requires us to model relative rates and carbon flux and understand how carbon flows through ecosystems. If we do this correctly and act with precise intention to leverage the relevant energy flows in our favor, we can achieve efficient, scalable carbon drawdown with significant life-generating benefits – clean air, water, food, soil, and built environments. We can also use the products of biological sequestration to launch new markets and wood-based construction technologies.
As expressed in the UN report:
Timing is critical. Near-term deployment of negative emissions technologies is necessary to prevent severe and functionally irreversible tipping points.
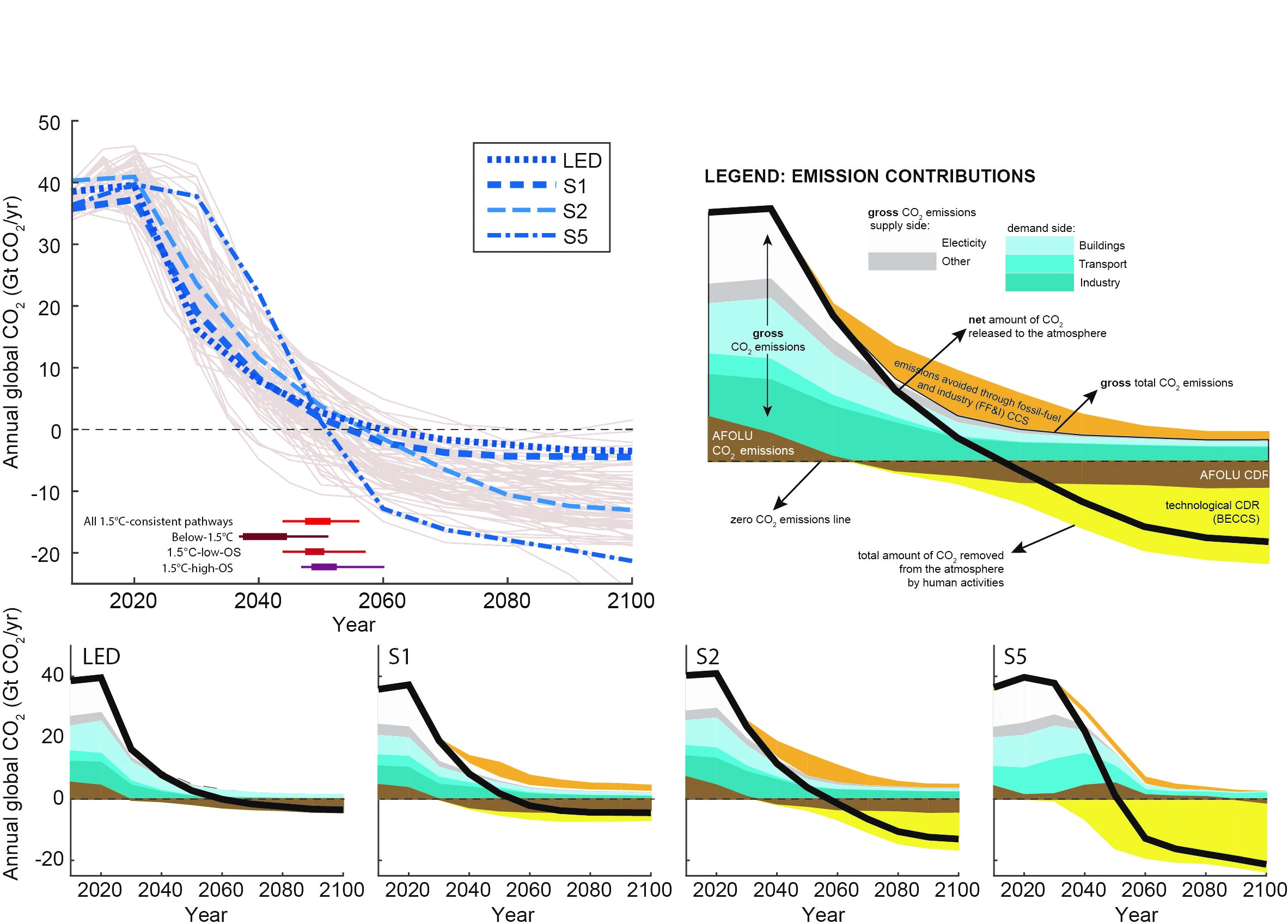
A close look at net-zero 2050 scenarios will reveal that many explicitly rely on massive scaling of photosynthetically produced biofuels for fossil fuel and wood burning replacement by 2050. We can unlock this same potential for carbon removal – if we monetize it appropriately. Why are we calling biological, non-permanent removal “low quality” when it is the dominant method by which we avoid crossing the 2-degree threshold under all realistic models?
Furthermore, the carbon removal method assumed under these models is BECCS (BioEnergy production with Carbon Capture and Storage). This is usually considered an engineered, rather than nature-based method (included in the IPCC illustration above as “technological CDR”), but relies on photosynthesis as the carbon drawdown mechanism. Direct air capture from the general atmosphere is not included as a significant drawdown mechanism by the IPCC, for reasons explored above in this post, the distinction is instead between BECCS and AFOLU (Agriculture, Forestry and Other Land Use). In BECCS, plant biomass (for example, switchgrass) is grown under intensive cultivation conditions for carbon capture, and is then burned to generate electricity, with chemical carbon capture devices installed on the exhaust gas stream to recapture emitted CO2, which is then injected underground. This is operationally identical to a CO2 capture system installed on a point emissions source. BECCS is attractive from the perspective of producing a useful energy resource (electricity) in addition to achieving net carbon drawdown, but there are inherent inefficiencies in capturing, emitting, and recapturing carbon. Most notably and often cited, BECCS would require a land area equal to the size of India to cultivate enough biomass to achieve sufficient drawdown by this method alone. This degree of expansion of cultivation is implicitly assumed in models which posit BECCS as our primary drawdown method.
It would be far more efficient from an energetic perspective to decarbonize electricity generation using solar, wind, and other renewables, rather than rely on BECCS. In this instance, photosynthesis enhancement, decomposition inhibition, and similar "engineered nature" approaches can fill the place of BECCS without the disadvantage of massively increasing the land area of the planet under intensive cultivation. This would instead equate to additional carbon capture in the AFOLU category.
More than 80% of scenarios in the IPCC’s Special Report on 1.5ºC overshoot the 1.5ºC temperature threshold before returning to these levels using large-scale carbon removal in the second half of the twenty-first century. This assumes a level of substitutability between emission reductions in the near-term and further-off removals, a notion that is rapidly being institutionalized in net-zero targets. Here we have an institutional framework to incentivize the funding of permanence at the expense of the scaling we need right now. While temperature overshoots might be reversible, this is not necessarily the case for other climate and geophysical dynamics.
When we trace the path of carbon through the biosphere, we are thinking in terms of the movement of energy in living systems. If we take as our goal maximizing the flourishing of the living system of the planet through enhancing large-scale biophysical processes, we can achieve goals and unlock opportunities that are not possible in a linear commodity exchange.