Snowball Earth II
From a crisis of near total extinction, life evolves the capacity to expand into larger forms of boundless diversity.
About 700 million years ago, Earth was plunged into an ice age of unimaginable proportions. Every continental shelf was frozen solid covered by ice 100 meters thick. Viewed from space, Earth would look like a giant snowball.

However, before we get to this dramatic climax, let’s dive into how we got there.
The time between Snowball Earth I and Snowball Earth II is sometimes called “The Boring Billion” Or, to be more technical, the "Proterozoic glacial gap," of over a billion years, separates the Huronian and Cryogenian glaciations. From space, Earth would appear calm, and the atmosphere had settled into an equilibrium that could well have persisted until the present.
For decades, scientists have questioned this lull. Why, given that eukaryotic algae appear in the fossil record early in the Proterozoic, did it take a billion years for them to form a sufficient carbon sink to again disrupt the global climate? Intriguing fossils from India and China suggest that the equilibrium between photosynthesis and respiration was again disrupted by increased metabolic integration in the photosynthesizers. In deceivingly quiet waters, a revolution was going on at the cellular level.
The eukaryotic algae had massive selective advantages in the Proterozoic oceans. For one, they incorporated cyanobacteria and aerobic bacteria into their cells as chloroplasts and mitochondria. Additionally, due to their novel ability for sexual reproduction, they not only reduced the consequences of DNA damage from oxygen, but they also accelerated their rate of evolutionary change. All the conditions were now present for a level of biotic integration never before seen on Earth: eukaryotic multicellularity.
1.6 billion years ago, relatively soon after the demise of the Gabonionta, fossils appeared in rocks that were deposited in a shallow tropical sea in what is now central India. While the Gabonionta did not clearly belong to any living group of organisms, these fossils are far less ambiguous. Named Rafatazmia chikatrootensis, after their place of origin, they form filaments of neatly stacked cells, intentionally joined together to form a single, larger organism that was anchored to the seabed. They are the first known multicellular red algae, very similar to the red seaweeds that still live on Earth today. And they were not alone in these seas; another species, Ramathallus, forms a frilly set of lobes where again, individual cells can be clearly seen.
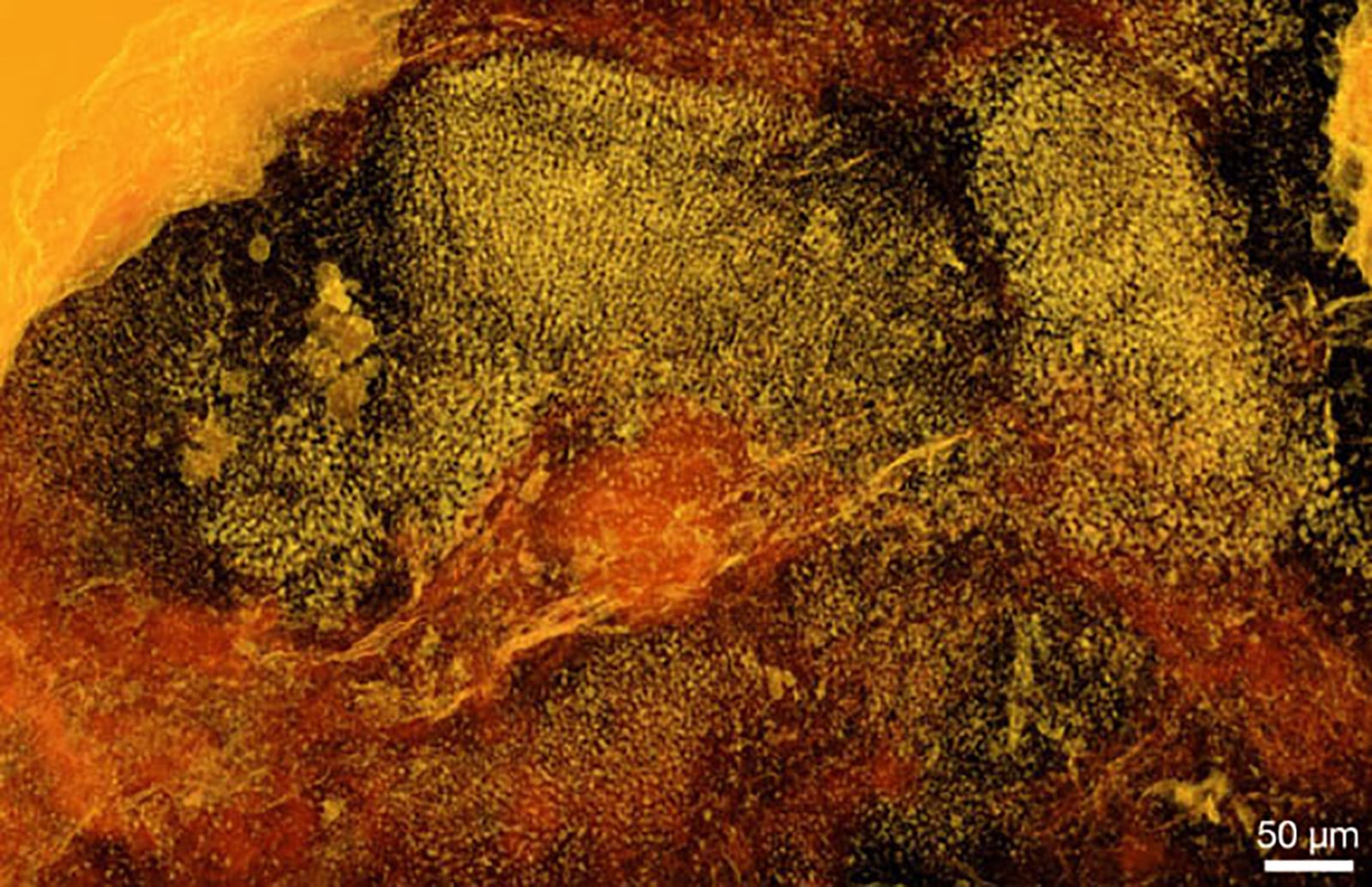
One billion years ago, the red seaweeds were joined by their green relatives, the direct ancestors of all living land plants. Fossils from China show the filamentous Proterocladus antiquus, with multiple thin branches and even a root-like anchoring structure, spreading across the continental shelves. This started the first period of the Neoproterozoic, the Tonian, and slowly undermined the equilibrium of the past 700 million years.
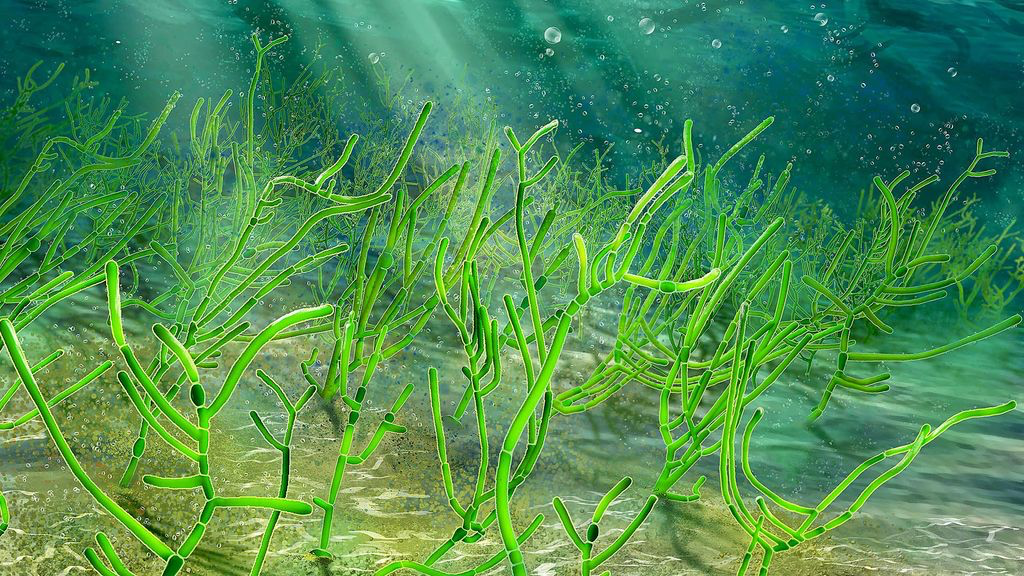
There were now multicellular eukaryotic photosynthesizers forming massive structures from fixed carbon, in the form of cellulose and other components of their cell walls. However, all known decomposers from the Proterozoic remained unicellular. They could secrete digestive enzymes on this biomass and, with the help of oxygen and their mitochondrial partners, extract energy using aerobic respiration. However, they could not mechanically process and digest the biomass directly. This was a world of seaweeds but no animals to eat them, a situation ripe to disrupt the carbon cycle.
By 800 million years ago, oxygen levels had long ended their slow decline and began to quickly accelerate. While destructive effects on life were not seen this time (with the experience of the biosphere with this gas), the accompanying carbon drawdown steadily converted the CO2 in the atmosphere into the body parts of seaweeds, which did not decompose efficiently, and again the planet began to cool.
This period also coincided with a particularly low solar irradiance and the breakup of the supercontinent Rodinia. This exposed gigatons of rock to the atmosphere and enhanced the removal of CO2 through weathering, along with a reduction in CO2-releasing eruptions at subduction zones. All of these factors would combine to make the Cryogenian ice age the most intense the Earth has ever seen.
There were multiple pulses of glaciation. The two most significant are the Sturtian (715 to 680 MYA) and Marinoan (650 to 630 MYA). Both of these glacial deposits are seen from what were then equatorial regions, showing that these were true Snowball Earths.
The Sturtian glaciation was so intense that the oceans again became anoxic, sealed under their ice cover, even though the atmosphere was rich in oxygen. This enabled iron sulfide to once again exist in their waters as it had back in the Archean. Periodically, the ice would crack and melt in places, allowing oxygen to enter and disrupt this echo of the Archean age. When this happened, the oceans again would rust and iron oxide would drop to the seabed, and as in the Archean, banded iron formations would form. The most recent banded iron formations known are from these dark and frigid ice-wrapped sulphidic seas.
The initial warming from the Sturtian glaciation was sudden and intense: enabled by slow volcanic CO2 rise, and triggered by a runaway melt process as exposed dark waters and land surface absorbed the sun's heat. The warming was so intense that the melting ice contributed unprecedented moisture to the atmosphere, and the warm humid atmosphere caused torrential global rainfall. This rain ran down over the suddenly ice-free continents, and the atmospheric CO2 dissolved in water reacted with the exposed rock to form carbonates, which were deposited in rock layers up to 30 meters thick. These cap carbonate deposits reflect an intensity of rainfall beyond human experience. It would have been as though the rainfall levels seen now in Seattle extended over the entire Earth, and persisted for thousands of years.
The same rain that caused the cap carbonates also resulted in massive river systems washing water rich in nitrogen, phosphorus and other nutrients into the oceans. These nutrients removed limiting factors to photosynthesis, and provided the seaweeds with the nutrients they needed to thrive and diversify globally, so much so that they would soon cause another global glaciation.
The period between the Sturtian and Marinoan glaciations, the Cryogenian glacial gap, while only lasting 30 million years, is of pivotal importance in the evolution of the biosphere. In these warm oceans, rich in nutrients and diverse multicellular algae, the heterotrophs would finally become durably multicellular. The earliest evidence of multicellular animals comes from this period. Additionally, during this time some of the green algae began to specialize for survival at the edges of the meltwater pools that were strewn across the continents. These would later form the ancestors of the land plants.
A third group of eukaryotes, decomposition specialists - the fungi - also appear at this time. Some of these would enter into cooperation with green algae, protecting them from exposure to the desiccating atmosphere inside their tissues, and anchoring them to the exposed rock. These cooperatives would become the lichens.
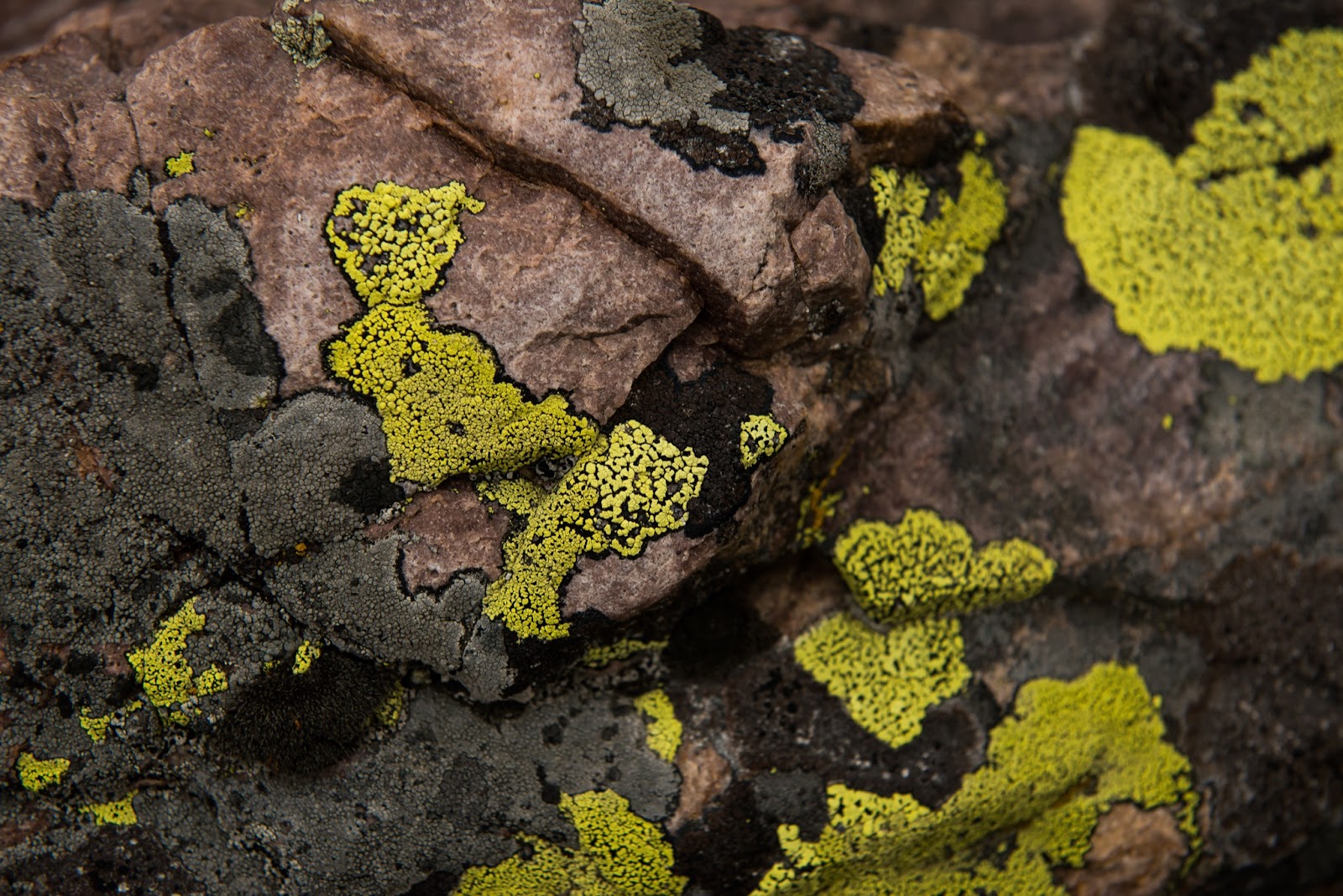
While all this ferment would later result in the Cryogenian being the last time the Earth froze to the equator, for now the algae still outpaced the decomposers. The initial result of the huge influx of nutrients was massive algal productivity, and another Snowball Earth: the Marinoan glaciation.
After another episode of banded iron formations and more cap carbonates, the final thaw exposed a changed world. Strange feather and quilt-like animals swayed in ocean currents and lay on the beaches, at first glance a little like the Gabonionta. These were the Ediacaran organisms, likely animals but not closely related to modern groups. The ancestors of the modern fungi were present, and the continents were beginning to support terrestrial life in the form of lichens and moss-like plants. Jellyfish swam through the oceans and sponges filtered bacteria and unicellular eukaryotes from the water column, feeding on them and returning their carbon to CO2. And in this warm world, a new kind of animal would soon evolve, fundamentally rebalancing the carbon cycle, and enabled by the now stably high concentration of atmospheric oxygen.

The decisive event that freed the planet from its vulnerability to global glaciation caused by the photosynthesizers is known as the Cambrian Explosion. The animal group that became ecologically dominant during this time are known as the bilaterians, after the pattern of symmetry of their bodies, with two sides, a head end, and a tail end, unlike the radial symmetry of a jellyfish or coral polyp.

Jellyfish are mostly predators. They contain other animals inside their bag-like stomach, and when done with digestion, release waste through the same orifice they used to consume their prey, which serves as both mouth and anus. While some corals and jellyfish host algae within their bodies and can photosynthesize, they are not herbivores. They do not consume bulk photosynthetic biomass as a carbon source.
In contrast, the bilaterians, the group to which humans and the majority of living animals belong, have both a mouth and an anus, so for them digestion can be an efficient continuous process, with the animal moving forward, eating, and passing the waste out behind it. This is also associated with the development of specialized organs such as image forming eyes and brains to process information and find food, and muscles to move the body of the animal toward it and push it into the mouth.
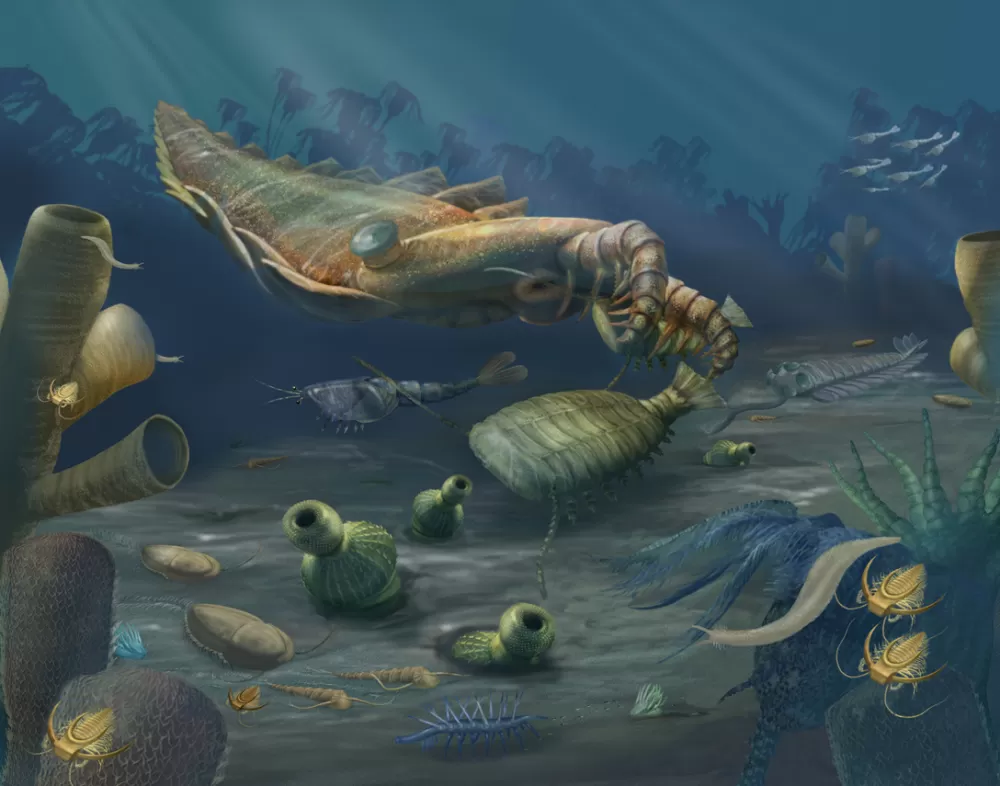
With the advent of bilaterian animals, the biosphere at last contained organisms that could fragment and decompose algal biomass at a rate similar to its creation, and was freed from global glaciation. Soon these bilaterian animals began to eat one another, and the prey developed hard carbonate shells to defend their soft bodies, while several forms of jaw developed amongst the predators. These animals included the ancestors of modern crustaceans, mollusks, fishes and many others. The quilt-like Ediacaran organisms were rapidly chewed up and tunneled into, and quickly disappeared, replaced by the small shelly fauna, and soon by spectacular fossil assemblages such as the Burgess Shale. This was the start of the era of globally prevalent macroscopic life, the Phanerozoic eon.
This post was part of our Deep Time Series. Continue reading the next post: Plants, Rocks, and Cascading Global Cooling